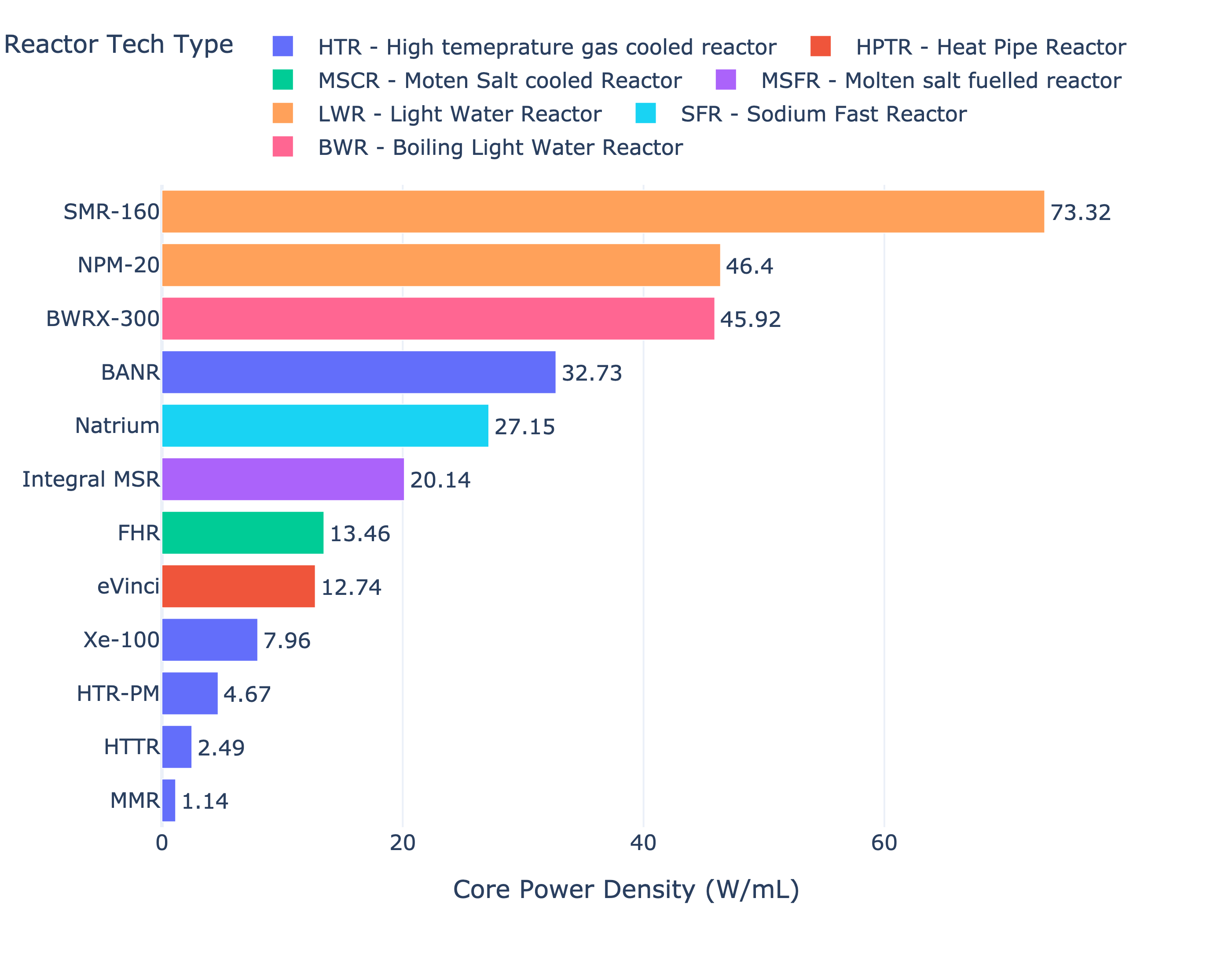
Advanced Fission Reactors
As a side-project, I collect and maintain information on upcoming nuclear energy extraction systems. Having it all in one place makes it useful for the public at large, policymakers, and scientists looking for a better understanding of the unfolding nuclear technology landscape.
There are now many designs, perhaps as many as 100 that are actively developed with more than a handful of people. To an outsider, these designs are all the same or arbitrarily different. Neither perception is true - there are important differences concerning cost and safety that should be more widely discussed.
Many of the topics and technologies discussed are in flux and there may be some delay in updating information to match new realities. Technology descriptions and information provided are based on publicly available information, subject to my interpretations. Anyone can send corrections, comments, or new information.
Are they Coming?
Advanced nuclear is already here. There are many advanced reactor designs worldwide at various stages of development. China and Russia are leading the way with completed demonstrations. Canadian and United States firms are playing catch up, many with significant challenges due to political cycles, manufacturing and construction decline, and other policy factors. In North America, some vendors claim 2026 demonstrations, but most are aiming for 2030.
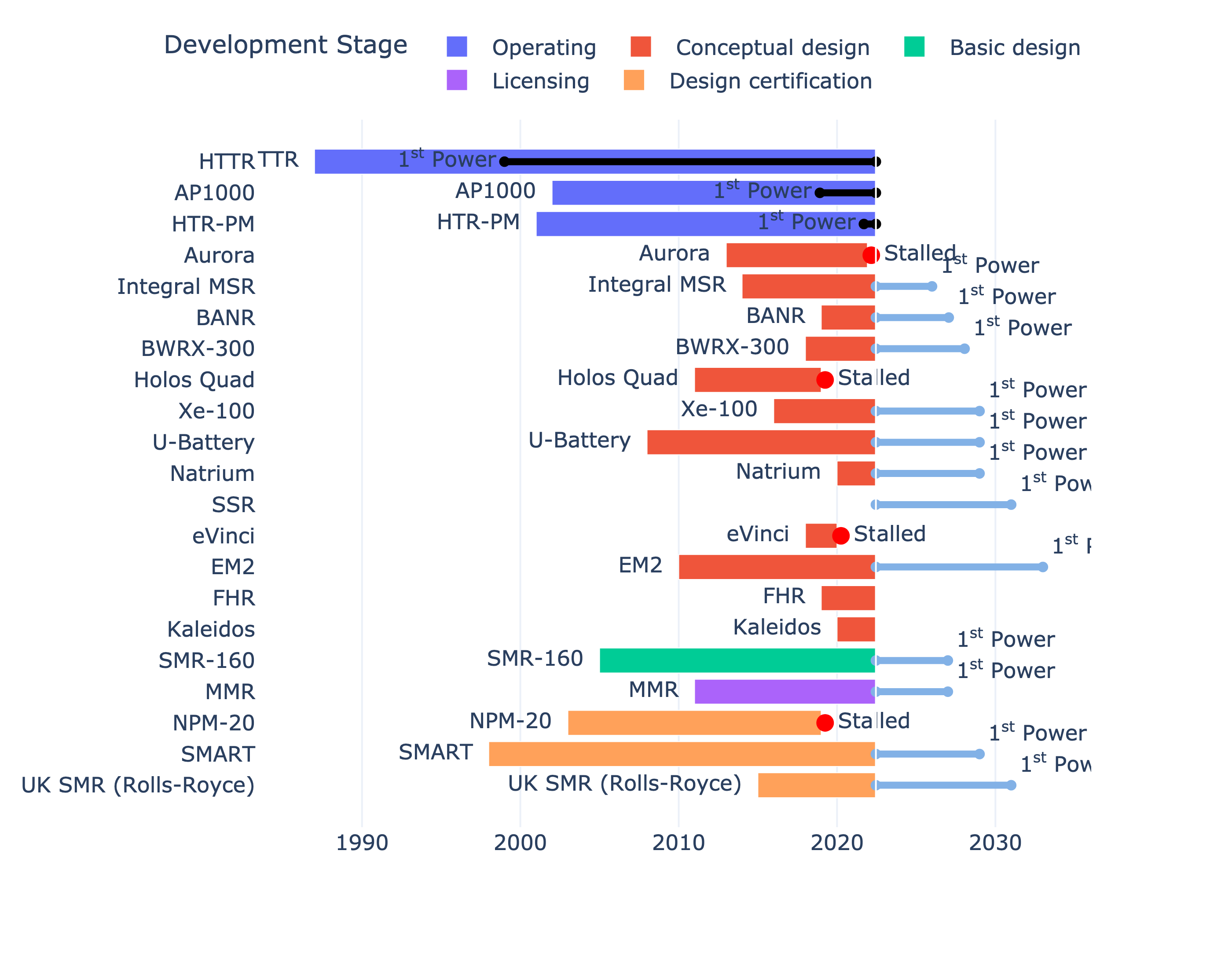
What makes a reactor "advanced"?
The vast majority of operating nuclear reactors are large reactors with ordinary fuel pellets, cooled by water, and producing gigawatts of power. These are the LWRs, BWRs, and CANDUs. "Advanced" refers to a deviation from these designs with the aim of improving cost, safety, or capability.
The simplest deviation is a reduction in size and power like the NuScale reactor, GE-Hitachi's BWRX-300, mPower, Holtec-160, or Rolls Royce SMR. These are just smaller water cooled reactors that should be easier to finance and build. This is vanilla flavored "advanced," barely deserving of the term. Even though they are very large and high power, Hualong One and AP1000 could also be considered "advanced" because they include some form of passive cooling systems that are not standard issue in the current fleet of water-cooled reactors.
A step further in "advanced" would be to use coolants other than water. This could be liquid sodium coolant like the Natrium reactor. Other coolants could be helium (High temperature gas-cooled reactors), molten salts, or liquid lead. There are various pros and cons to each coolant, but generally the designers seek to replace the corrosion, hydrogen generation, and pressure issues associated with water. Using coolants other than water can reduce the powerplant's complexity significantly.
Even more advanced would to use new fuel forms that improve safety and fuel utilization. This could the TRISO fuel used in gas-cooled reactors and FCM/TRISO used in USN's MMR. These fuels dramatically improve accident tolerance, allow for higher fuel use efficiency, and allow the reactors to deliver higher temperature heat. There are also fuels and reactors that aim to reprocess the used fuel into new fuel as in liquid fuel reactors like Terrestrial Energy.
For a critical perspective on advanced nuclear, see Ed Lyman's report: 'Advanced' Isn't Always Better.
How big are they?
The proposed reactors are of many sizes and power levels. It is important to understand that power and size are not always related. Traditional nuclear and coal plants have been about 3000 MWth. Recent designs will produce between 3 MWth and 3,400 MWth. Only about 1/3rd of that power ends up as electricity, enough for about 1,000 and 1 million people, respectively. The overall trend is towards smaller plants in both size and power. These are more easily financed and built.
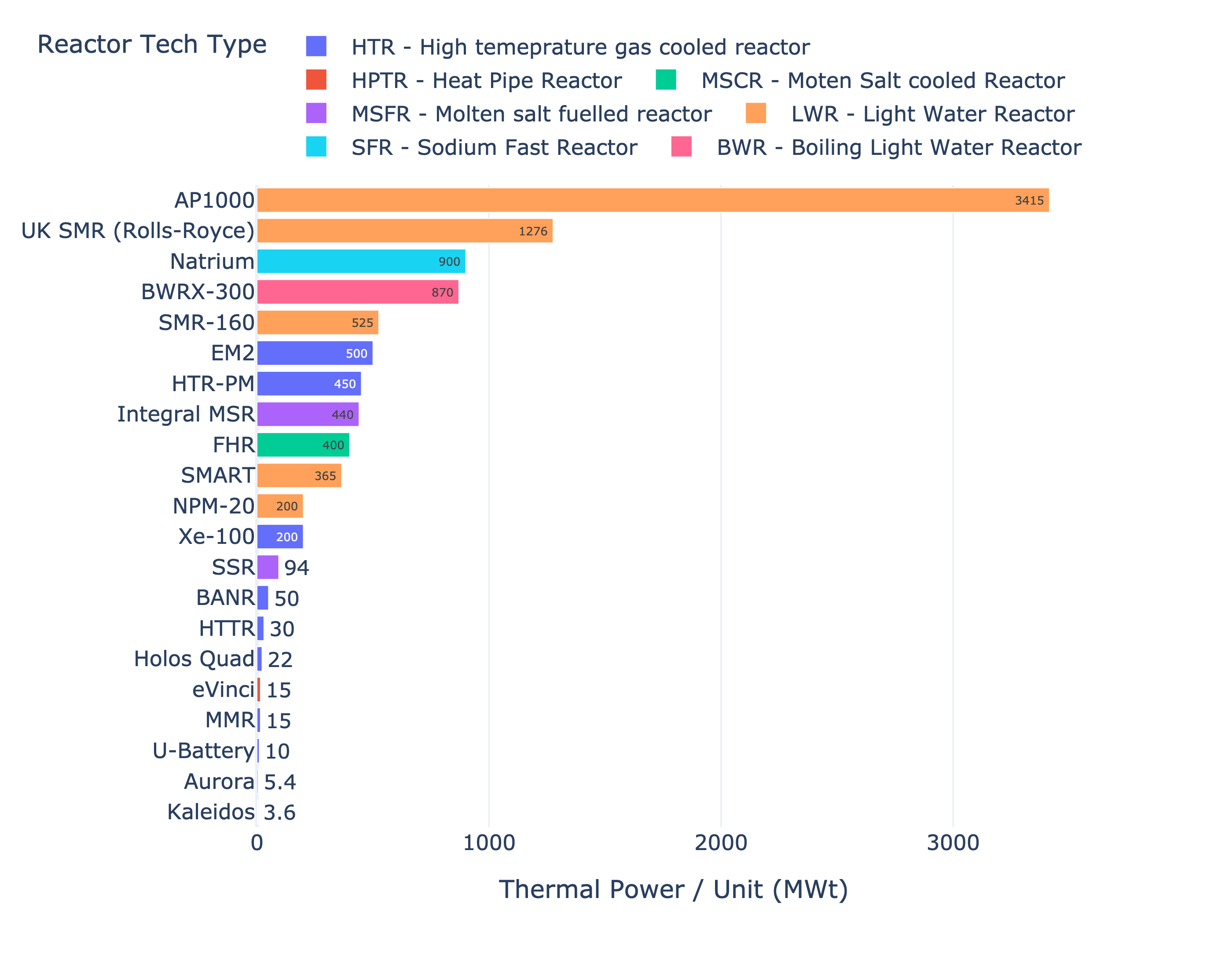
Dumb Reactor Nomenclature: SMRs and MMRs
Reactor vendors and policymakers like to brand designs with new names for various reasons. The vendors like to lay claim to ideas with special new names - staking the territory and making it easier to talk about significantly different reactor types. Policymakers like using special names because it allows for nuclear rebranding and targeted funding language.
Since the late 2000s, the "Small Modular Reactor" (SMR is now the stock ticker for Nuscale) has represented reactors that are roughly 5-10x smaller in thermal power level than conventional gigawatt scale reactors. The idea has been that smaller reactors will be more cheaply built in factory environments and with lower investment risk. IAEA has a long article.
In 2011, the "Micro Modular Reactor" (MMR is trademarked by Ultra Safe Nuclear) was conceived to represent even lower power reactors than SMRs. The design emerged right after Fukushima with a new attention to safety - in this case using accident tolerant fuels that wouldn't melt at Fukushima-like temperatures and going to very lower density in the reactor. Going to such low power levels pushes the factory advantages even further and allows for a paradigm shift in safety in which the reactor does not use safety systems to avoid meltdowns or catastrophic accidents. Since "MMR" was trademarked reactor vendors who have followed Ultra Safe Nuclear into the lower power design space have started using the term "micro reactor" for power levels less than about 50 MWth. The term has been applied to non-economic designs like Idaho's MARVEL and the US Department of Defense's container reactors which, while low power, cannot be reasonably compared to MMR-like reactors on a cost or safety basis. The term "micro reactor" means basically nothing other than low power level, especially when brandished by engineers, reporters, and bureaucrats.
Since 2020, the term "fission battery" has been circulating in academic settings to define what is essentially the MMR – reactors that are small, transportable, and inherently safe with a suite of benefits for operations and safety. In practical terms, a "fission battery" is just another word for "micro reactor." The academics are finally catching up to the industry designs and making note of the potential radical benefits of MMR-like reactors.
As described in the academic literature, core tenets of the "fission battery" are:
- Economic – Cost competitive with other distributed energy sources (electricity and heat) used for a particular application in a particular domain. This will enable flexible deployment across many applications, integration with other energy sources, and use as distributed energy resources.
- Standardized – Developed in standardized sizes, power outputs, and manufacturing processes that enable universal use and factory production, thereby enabling low-cost and reliable systems with faster qualification and lower uncertainty for deployment.
- Installed – Readily and easily installed for application-specific use and removal after use. After use, fission batteries can be recycled by recharging with fresh fuel or responsibly dispositioned.
- Unattended – Operated securely and safely in an unattended manner to provide demand-driven power.
- Reliable – Equipped with systems and technologies that have a high level of reliability to support the mission life and enable deployment for all required applications. They must be robust, resilient, fault tolerant, and durable to achieve fail-safe operation.
What are differences between designs?
There are many ways to peel a potato, and there are many ways to produce nuclear energy. The principal differences between designs are the choice of fuel, coolant, reactor size, and power level for the reactor. These design choices matter because they affect the economics and safety of the nuclear reactor.
Reactor vendors should aim to minimize costs while maintaining sufficiently low accident risk. This is often not the case – reactor designers will push for their own pet ideas, policymakers will support projects in their jurisdiction, financial managers will support the short-term highest profitability concepts, and technical reviewers will support the most credible and lowest risk concepts (i.e. the most mundane ideas and companies that have already tried and failed in the past).
In any case, today there a few general families of reactor technologies that you can read more about elsewhere. Any discussion of the technologies invariably degrades into a partisan debate about the claimed advantages and disadvantages. The debates are far from settled and reach their highest pitch in the "expert class" of nuclear engineers at the universities and companies.
The technical aspects and debates should not prevent non-technical people from forming a view. After all, they will be living around reactors and subject to its risks. We cut through the debate by looking at some baseline metrics of nuclear energy systems.
Dumb Reactor Nomenclature: Generation I, II, III, IV
Safety
Power Density
Lower power densities are more manageable during accidents, and all other things being equal, translate into safer designs.
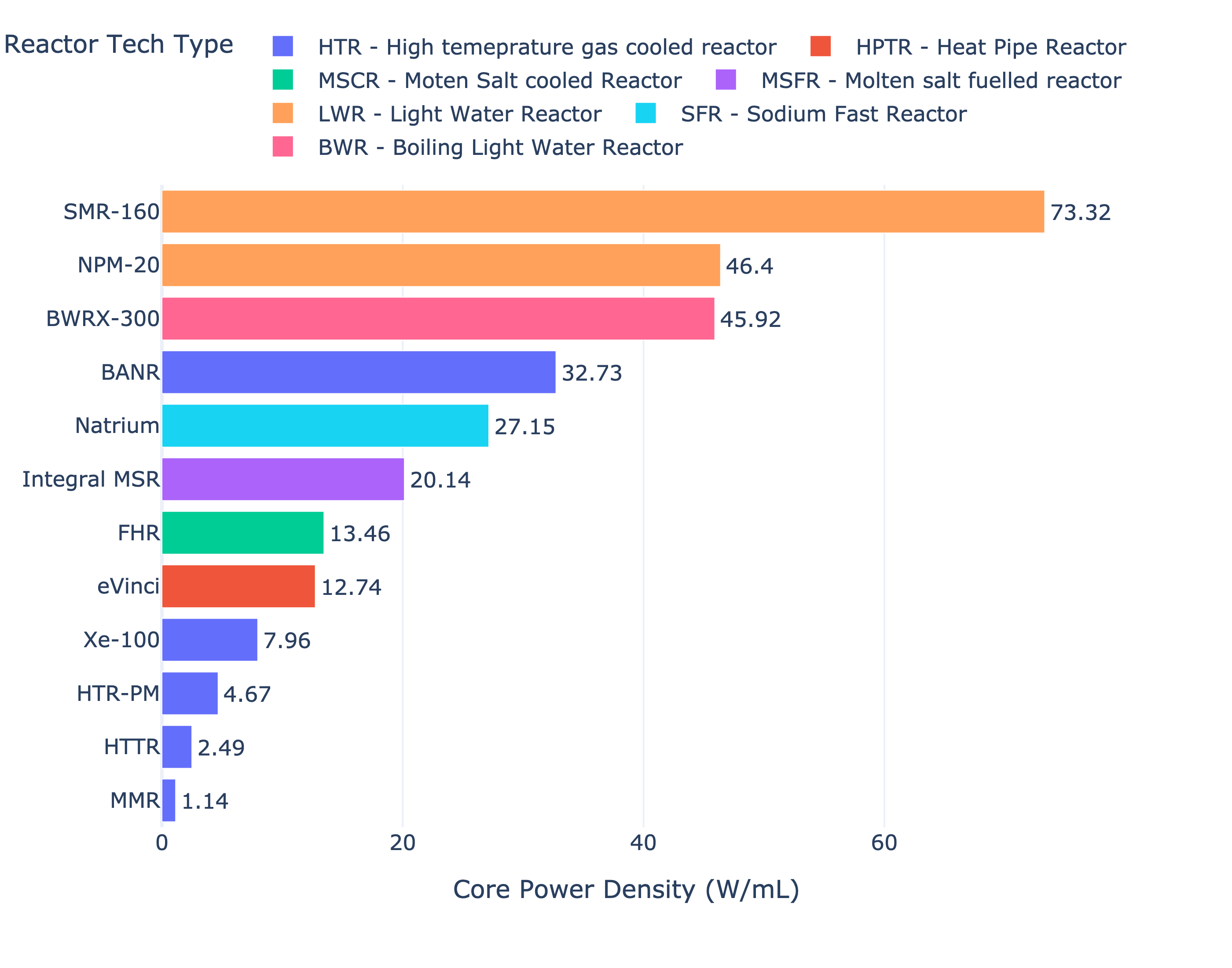
Surface Area to Power Ratio
Values were calculated using industry knowledge and publicly available information. Higher surface area to power ratio translates into a safer reactor as heat can be more easily dissipated by passive, solid state heat transfer.
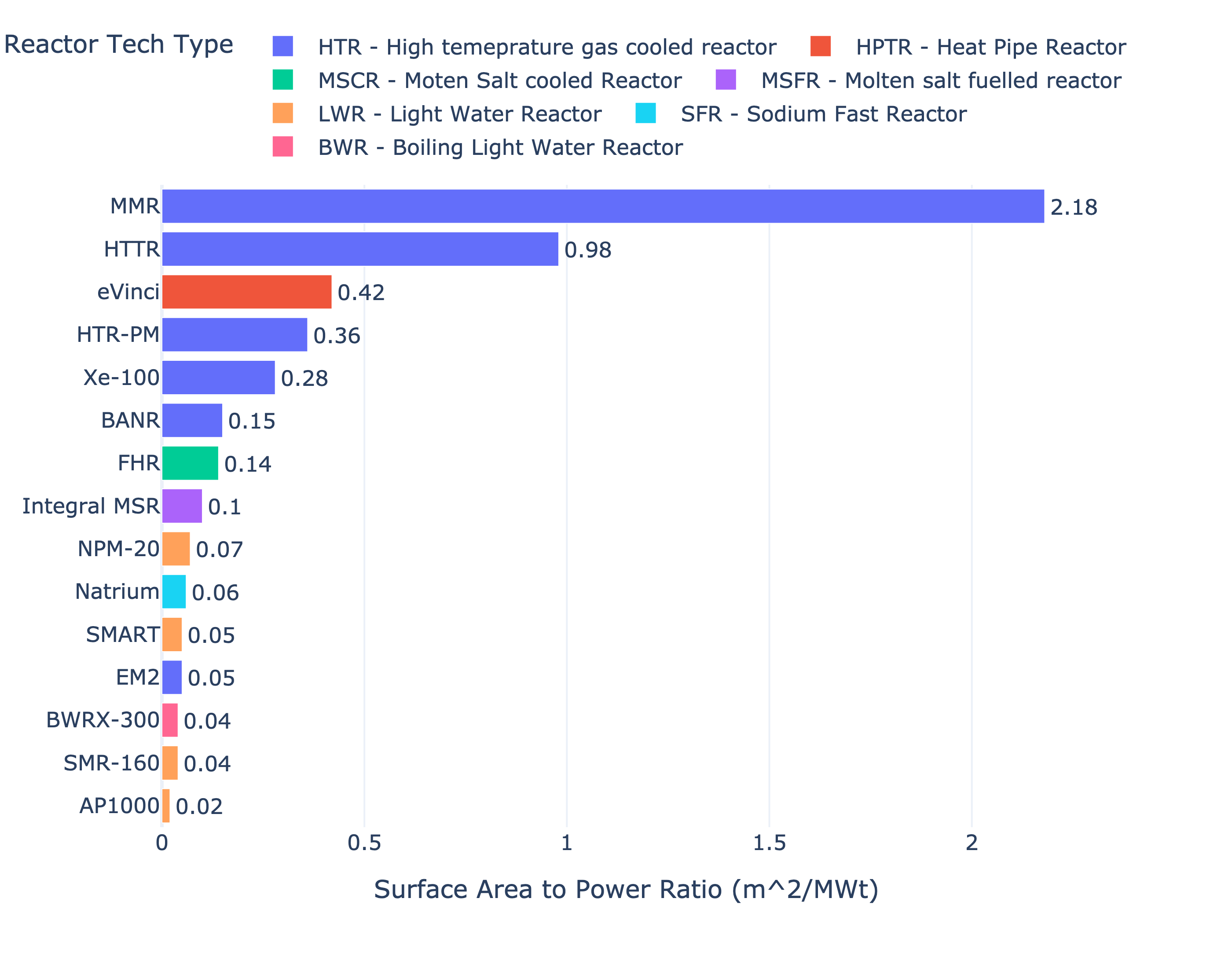
Enrichment
HALEU greater than 10% enrichment does not exist in the US for civilian uses as of 2022, and significant quantities are unlikely to be available for demonstration projects before 2030, let alone commercial rollouts. enrichments less than 10% are more widely available today. Lower enrichments designs unlock several benefits with a cascade of knock-on advantages. Lower enrichment fuel drastically improves the risk profile for technology demonstration and deployment. Supply chains do not have to be created from scratch and favorable regulations and facility requirements are allowed for lower enrichment fuel, which will directly impact fuel fabrication and reactor manufacturing costs. Finally, lower fuel enrichment reduces proliferation risk because it takes more energy and effort to enrich to weapons grade material.
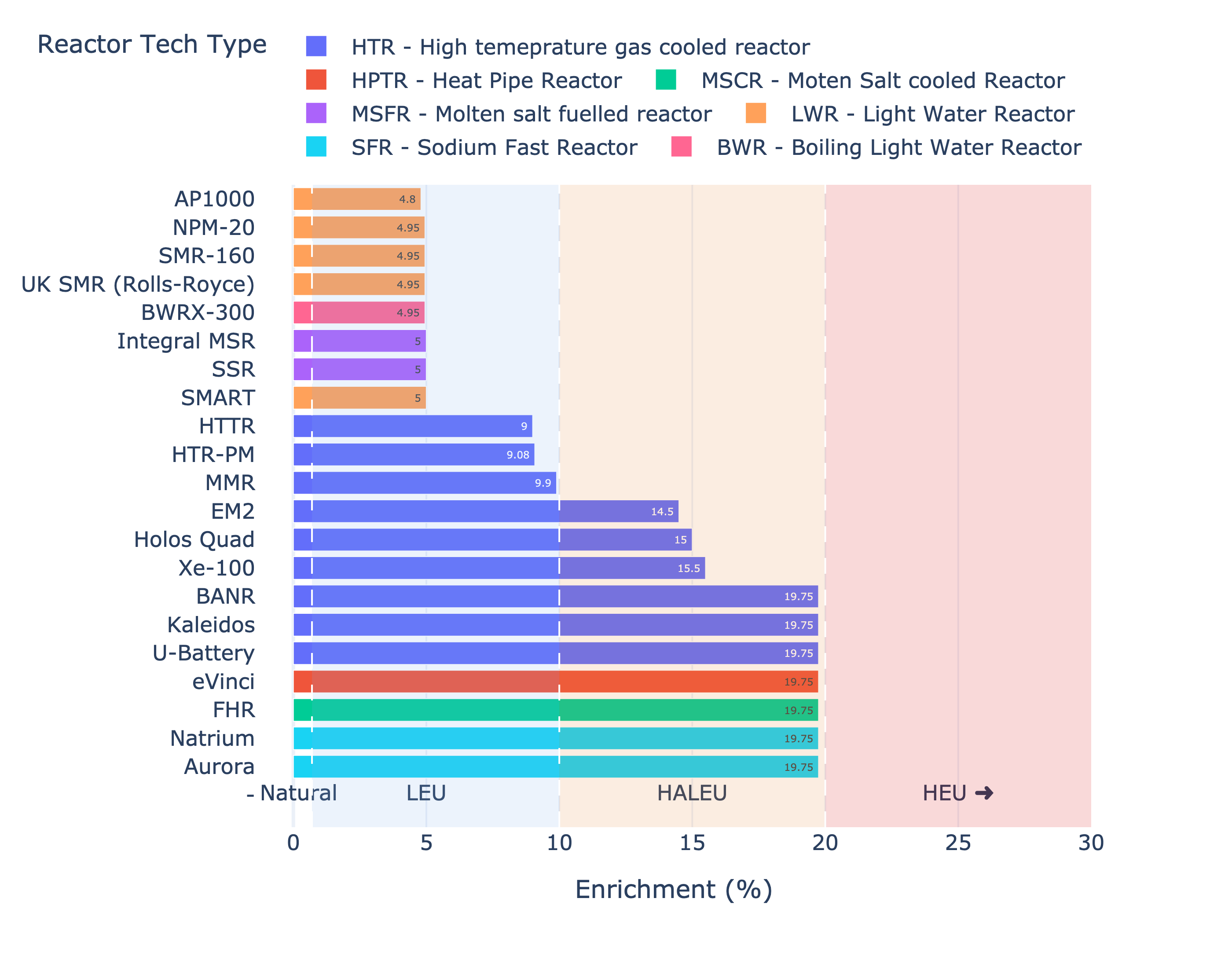
Fuel, Moderator, and Coolant
A reactor technology is basically the choice of fuel, moderator, coolant, size, and power rating. Each particular combination will require particular analysis and has pros and cons. The table below lists some of the most prominent designs. There is quite a bit of diversity here. If you want to start a new nuclear company, just pick a new combination!
A good designer will consider various hazards of a nuclear power plant: the nuclear decay heat, the chemical compatibility of the components, the risk of operator error or terrorist attack, pressure hazards, theft of nuclear material etc.
Reactor | Fuel | Moderator | Coolant |
---|---|---|---|
USNC MMR | FCM TRISO | Graphite | Helium |
USNC PYLON | FCM TRISO | Hydride | Helium |
Westinghouse eVinci | Uranium Metal | Graphite | Heat pipes |
X-Energy Xe-100 | Filled Pebbles TRISO | Graphite | Helium |
BWXT BANR | Graphite TRISO | Graphite | Helium |
BWXT PELE | Graphite TRISO | Water | Helium |
Kairos FHR | Hollow Pebbles TRISO | Graphite | Fluoride salt |
Terrestrial Integral MSR | Liquid UF6 + UF4 in NaF/RbF | Graphite | Fluoride salt |
NuScale NPM-20 | UO2 Pellets in Zircalloy Clad | Water | Light water |
TerraPower Natrium | UZr Metal fuel | Fast Reactor | Sodium |
GE-H BWRX-300 | UO2 Pellets in Zircalloy Clad | Water | Light water |
Holtec SMR-160 | UO2 Pellets in Zircalloy Clad | Water | Light water |
Oklo Aurora | UZr Metal fuel | Fast Reactor | Heat pipes |
Moltex SSR | Liquid UF6 + UF4 in NaF/RbF | Fast Reactor | Fluoride salt |
GA EM2 | Graphite TRISO, SiC Cladding | Graphite | Helium |
MGHTR | Graphite TRISO | Graphite | Helium |
Radiant Kaleidos | Graphite TRISO | Graphite | Helium |
HTTR | Graphite TRISO | Graphite | Helium |
CNNC HTR-PM | Filled Pebbles TRISO | Graphite | Helium |
Database
Working on a database display and easy download.
Further Reading
Books
- Weinberg, "The Second Nuclear Era: A New Start for Nuclear Power"
- Weinberg, "Science and Trans-Science"
Papers
- Weinberg, "Advanced Reactors, Passive Safety, And Acceptance Of Nuclear Energy!"
- Lyman, "'Advanced' Isn't Always Better."
- Li, "A New Paradigm for Civil Nuclear Energy."
- Weinberg, "Science and Trans-Science."
- Lidsky, "The Trouble With Fusion."
- Reutler, "Advantages Of Going Modular In HTGRs."
- Fukaya et al., "Conceptual Design Study of a High Performance Commercial HTGR for Early Introduction."
Useful Links
- Wikipedia List of Reactors
- List of SMRs
- IAEA Database
- World Nuke Map
- Nuclear Thefts
- whatisnuclear.com
- nuclear-power.com
- Nuclear Innovation Alliance
There are some descriptive lists from IAEA and elsewhere (IAEA (archive), Columiba, IAEA Database, IAEA Reports, etc), but these tend to follow the official narrative, without making use of information obtained in talks and conferences, and without technical extrapolation or interpretation. They lack useful technical details like reactor dimensions, fuel types, and any other derivative metrics like power density.